INTRODUCTION
According to the World Health Organization, age-related macular degeneration (AMD) is the third leading cause of visual impairment worldwide resulting in blindness with a prevalence of 8.7%. AMD is also the leading cause of visual impairment among elderly in industrialized countries. It is likely that AMD prevalence increases in proportion to the increase of the elderly population. Epidemiological data from recent years indicate that the AMD incidence increased from 5% to 27%(1).
In neovascular AMD, there is a subretinal neovascular membrane and exudation results in intraretinal edema. After treatment with ranibizumab, retinal edema decreases, leading to retinal thinning. Recent studies have suggested that other assessment methods should be used in neovascular AMD, including optical coherence tomography (OCT) for qualitative and quantitative image analysis and accuracy improvement by characterizing the retinal morphology impairment. The major OCT limitation is the difficulty to perform the tomography of the very same retinal region before and after treatment in order to make an appropriate comparison(2).
Multifocal electroretinography (mfERG) provides a topographic map of retinal function using parameters that ensure a proper evaluation of the photopic response. The objective measurements provided by mfERG enable quantification of responses to light from photo-receptors, second order neurons, and inner retinal neurons. mfERG enables simultaneous measurements of responses originating from localized areas in the central and peripheral retina. In this way, mfERG differs from full-field electroretinography (ffERG), which records the global response of the whole retina. Information provided by mfERG for specific retinal areas may assist in explaining functional losses observed by psychophysical methods and complement the structural evaluation provided by ophthalmological observations and OCT imaging. Furthermore, mfERG may reveal the occurrence of retinal dysfunction even when ophthalmological observation, OCT imaging, and psychophysical evaluation suggest little or no impairment of the visual system(3).
One of the few studies using mfERG to evaluate neuroretinal function after the treatment of AMD with ranibizumab described stabilization or improvement of visual acuity and reduction of macular thickness after treatment, but also a reduction in mfERG amplitude in patients compared with that in controls(4). The authors suggested that edema reduction could lead to visual acuity improvement, but the drug may also cause tissue hypoxia leading to deterioration of retinal function(4).
Most studies on the use of ranibizumab for the treatment of neovascular AMD evaluated patients with relatively good vision (20/3020/150)(5-8). The objective of the present study was to use mfERG to study patients with advanced neovascular AMD (visual acuity 20/100 or worse) in order to determine if retinal function improves after ranibizumab treatment.
A short communication of the results of this work was presented in the Annual Meeting of the Association for Research in Vision and Ophthalmology (ARVO)(9).
METHODS
This interventional case series was performed in the Núcleo de Medicina Tropical and Hospital Universitário Bettina Ferro de Souza, both in Universidade Federal do Pará. We evaluated 17 patients (23 eyes) diagnosed with advanced neovascular AMD; two eyes were excluded because they had a visual acuity better than 20/100. Therefore, a total of 21 eyes with a visual acuity ≤20/100 (advanced neovascular AMD) were evaluated. We excluded patients with cataract opacity because this condition prevents reliable OCT imaging and mfERG recording.
Three intravitreal injections of anti-angiogenic ranibizumab were administered at 1-month intervals. OCT and mfERG (103 hexagons) were performed before the first injection (D0) and at 30, 60, and 90 days after injection (D30, D60, and D90, respectively). Macular OCT (fast macular thickness map, 6 mm) was performed at 10-µm axial resolution with Stratus OCT 3.0 (Carl Zeiss Meditec, Jena, Thuringia, Germany)(10). Total macular volume measurements were made using the default software provided by the device manufacturer.
The mfERG was performed with the Veris System 6.010 (Electro-Diagnostic Imaging, EDI, Redwood City, CA, U.S.A.), which was used for stimulation, recording, and data extraction. We used a high spatial resolution (1280 × 1024 pixels) and temporal resolution (60 Hz) FMS III microdisplay (EDI) to present a black and white stimulus for the patient’s eye. The stimulus was composed by an array of 103 hexagons covering 45° squared visual angle. The hexagons were scaled considering the changes in retinal cone density with eccentricity in order to elicit a uniform mfERG across retinal areas at different retinal eccentricities. The luminance modulation of each hexagon was driven by a complete cycle of an m-sequence of 214-1 elements. The same m-sequence was used to control luminance modulation of all hexagons, but the starting point of the m-sequence reading for each hexagon differed. One m-state controlled hexagon luminance at 200 cd/m2 (flash period), while another m-state switched off hexagon luminance to a residual screen luminance of 0.01 cd/m2 (non-flash period). The base period of the m-sequence reading was 16.6 ms. A red cross (1° of visual angle) was used in the center of the hexagon array as a fixation mark. An infrared camera (EDI) was used for eye movements monitoring. The recording duration lasted for approximately 4 min and 33 sec. The retinal activity was recorded by a corneal Dawson-Trick-Litzkow (DTL) electrode (active electrode) and surface electrodes that were used as reference (placed at the temporal canthus of the tested eye) and ground (placed at the center of the forehead) electrodes. The analog signal was amplified × 50,000, sampled at 960 Hz, and on-line filtered between 10 Hz and 300 Hz with a differential amplifier Model 15LT (Grass, Quincy, MA, U.S.A.). The analog signal was digitized using an acquisition board (PCIE series, National Instruments, Austin, TX, U.S.A.). The peak-to-baseline amplitude and implicit time of the first negative component (N1) and first positive component (P1) components were measured. The analysis was made in three different annular retinal areas: R1, which was composed by hexagons corresponding to the central 8° of the retina; R2, which was composed by hexagons located between 8° and 24° of eccentricity; and R3, which was composed by hexagons located between 24° and 45° of eccentricity. The average of the data from each region was obtained. All electrophysiological procedures were performed according to the guidelines of the International Society for Clinical Electrophysiology of Vision (ISCEV)(11).
A sex- and age-matched control group of 21 eyes was also evaluated using OCT and mfERG. We compared the results from OCT and mfERG patients with the control group using one-way ANOVA followed by Tukey’s post-hoc test (α=0.05).
All patients were studied according to the tenets of Declaration of Helsinki. Institutional approval was granted by the Committee for Research Ethics of the Núcleo de Medicina Tropical (Protocol #027/2010). Informed consent was obtained from all patients.
RESULTS
Patients included 12 women and 5 men, 70.76 ± 2.24 years old. Their visual acuities (logMAR ± standard error) at D0 and D90 were 1.75 ± 0.13 and 1.91 ± 0.14, respectively (P=0.28).
OCT performed prior to the treatment revealed an increased macular thickness probably due to intraretinal edema. We speculated that the increased thickness of the retinal pigment epithelium-choriocapillaris complex (RPE-CC) was compatible with the choroidal neovascular membrane. The temporal evolution of the retinal thickness was as follows: D0=8.44 ± 0.35 mm, D30=7.13 ± 0.22 mm, D60=7.16 ± 0.24 mm, and D90=7.23 ± 0.28 mm. Subjects without AMD had 6.52 ± 0.09 mm. Follow-up OCT examination showed a significant reduction in macular volume when D0 was compared with follow-up examination (P<0.01 one-way ANOVA, Tukey’s post-hoc test), but persistence of the increased retinal thickness compared with controls (Figures 1-2).
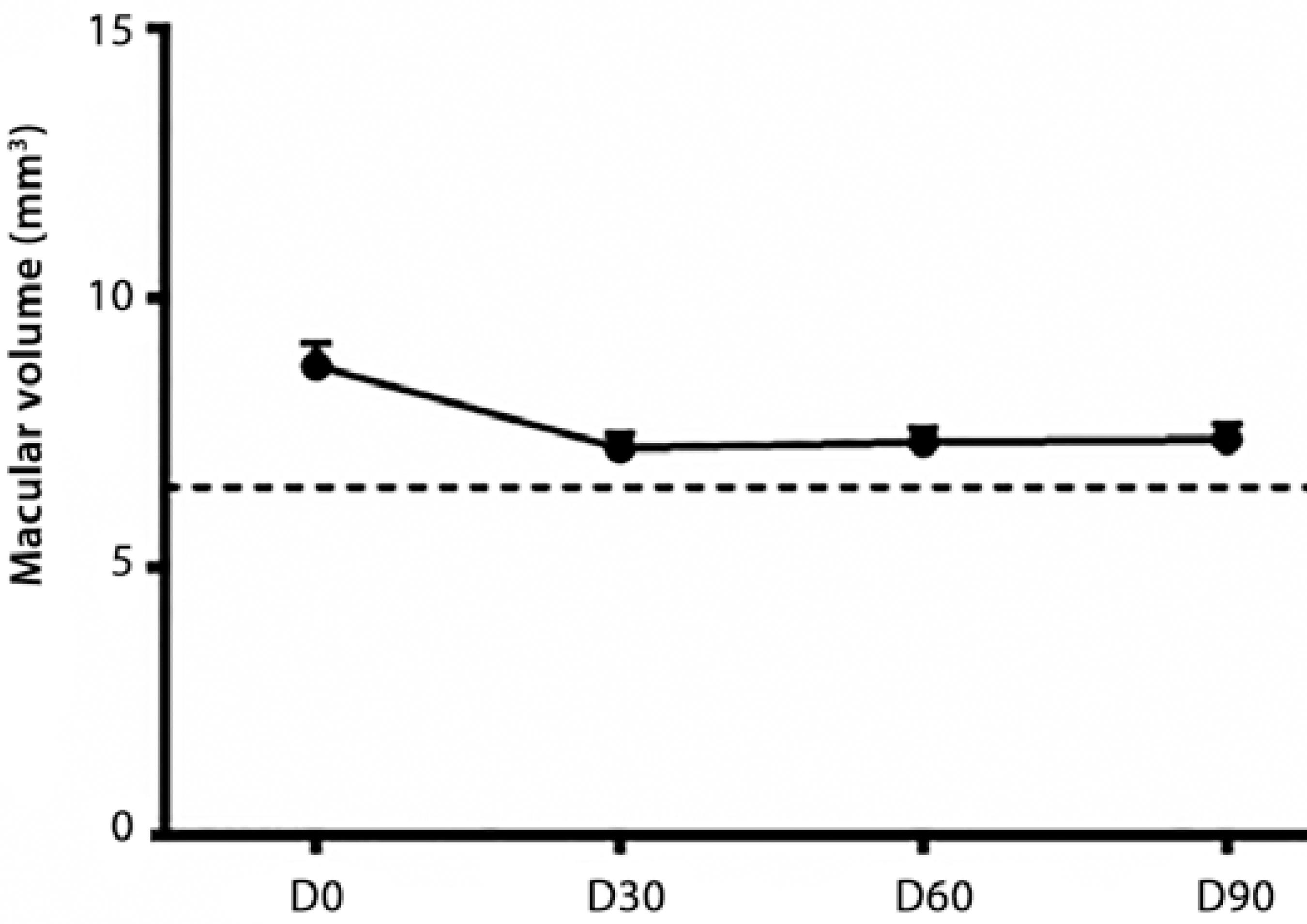
Figure 1 Optical coherence tomography (OCT) of macular volume of controls (dashed line) and patients (D0, D30, D60, and D90).
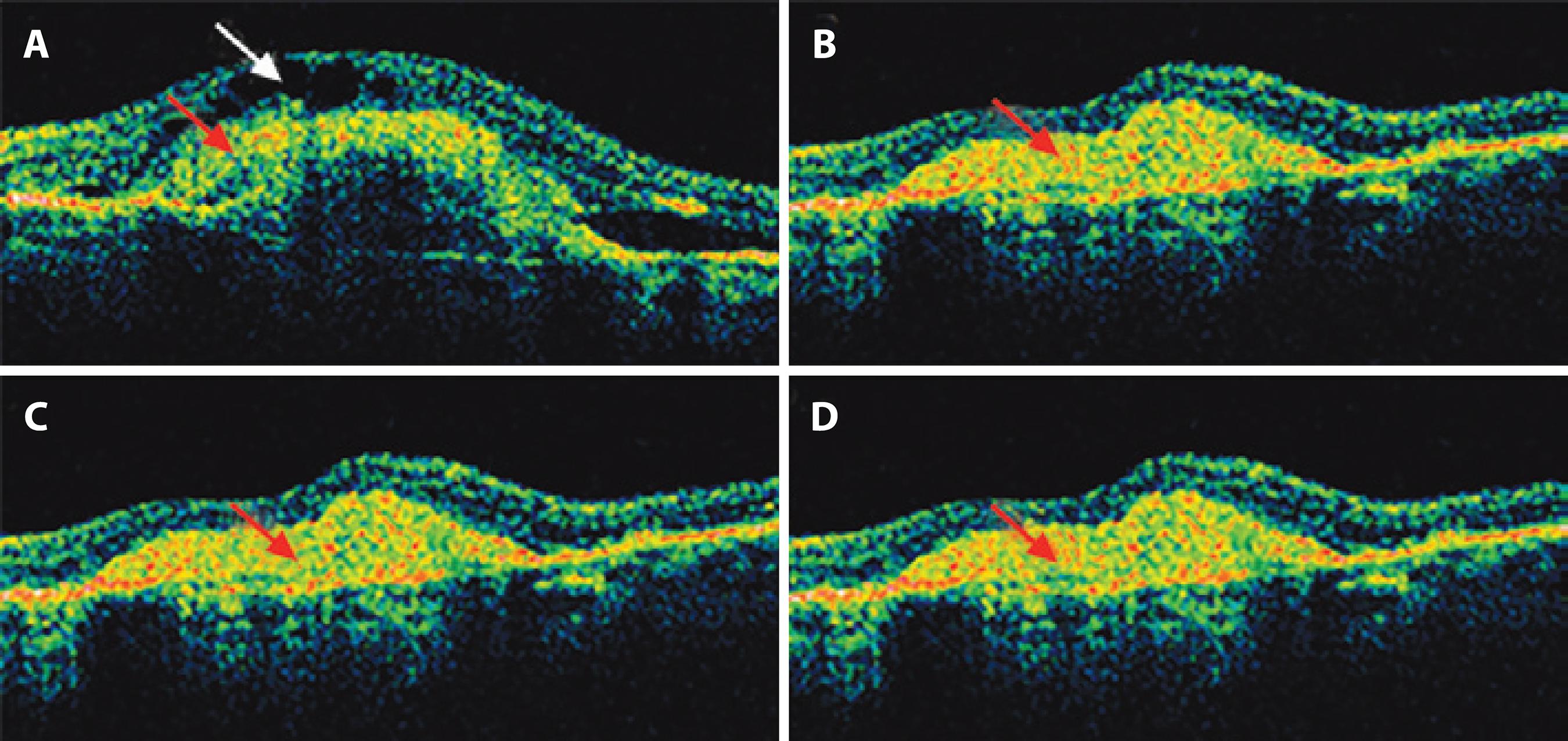
Figure 2 Optical coherence tomography (OCT) of patients at (A) D0, (B) D30, (C) D60, and (D) D90. An increased thickness of the inner layers of the macula area was seen at D0 due to intraretinal fluid (white arrow) as well as increased thickness of the retinal pigment epithelium-choriocapillaris complex (RPE-CC) (red arrow). Intraretinal edema was reduced at D30, D60, and D90 (P<0.01, ANOVA, Tukey’s test), but the RPE-CC (red arrow) resulted in a subretinal scar.
The results were grouped in three annular retinal areas for mfERG analysis: R1 (central area, 0°-8°), R2 (parafoveal area, 8°-24°), and R3 (peripheral area, 24°-45°). In each of these rings, we analyzed N1 and P1.
Prior to treatment (D0), N1 amplitudes in R1 did not differ among patients and controls, but after the first injection (D30), the amplitude was significantly reduced (P<0.01). This difference decreased 2 months and 3 months after the first injection (D60 P<0.01, D90 P<0.05, one-way ANOVA, Tukey’s post-hoc test; Figure 3 A). In R2, the N1 amplitude was significantly reduced in patients compared with that in controls over the entire testing period (P<0.01, one-way ANOVA, Tukey’s post-hoc test; Figure 3 A). In R3, the N1 amplitude was significantly reduced at D30, D60, and D90 compared with that in controls (P<0.01, one-way ANOVA, Tukey’s post-hoc test; Figure 3A).
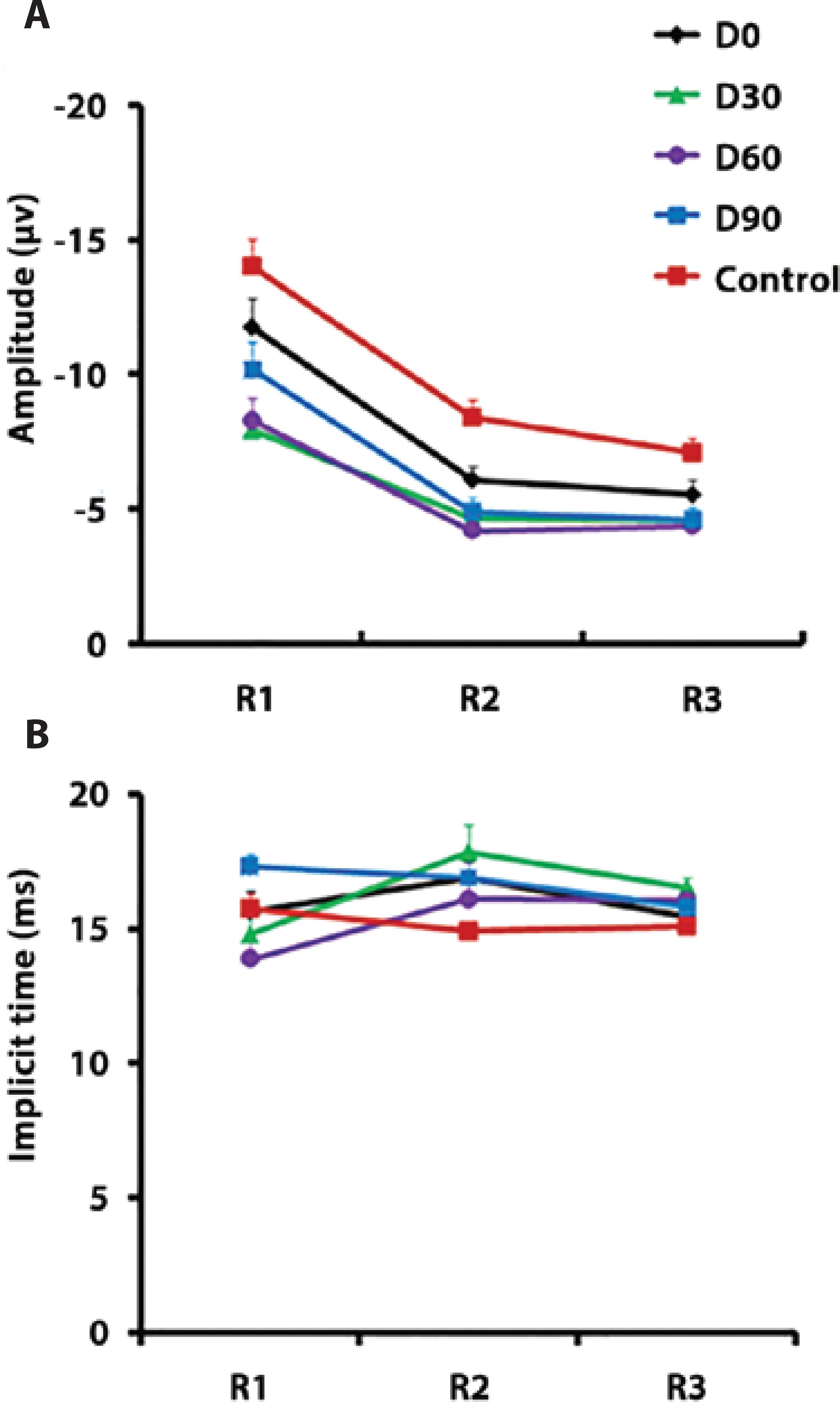
Figure 3 (A) Amplitude and (B) implicit time of the N1 component of the multifocal electroretinogram (mfERG).
In R1 and R2, N1 implicit time was not significantly different in patients and controls (Figure 3 B). In R3, N1 amplitude was delayed at D30 compared with that in controls (P<0.05, one-way ANOVA, Tukey’s post-hoc test; Figure 3 B).
Prior to treatment (D0), P1 amplitudes in R1 and R2 did not differ between patients and controls, but the amplitude was significantly reduced in patients at D30, D60, and D90 compared with that in controls (P<0.01, one-way ANOVA, Tukey’s post-hoc test; Figure 4 A). P1 amplitude in R3 did not differ between the two groups (Figure 4 A).
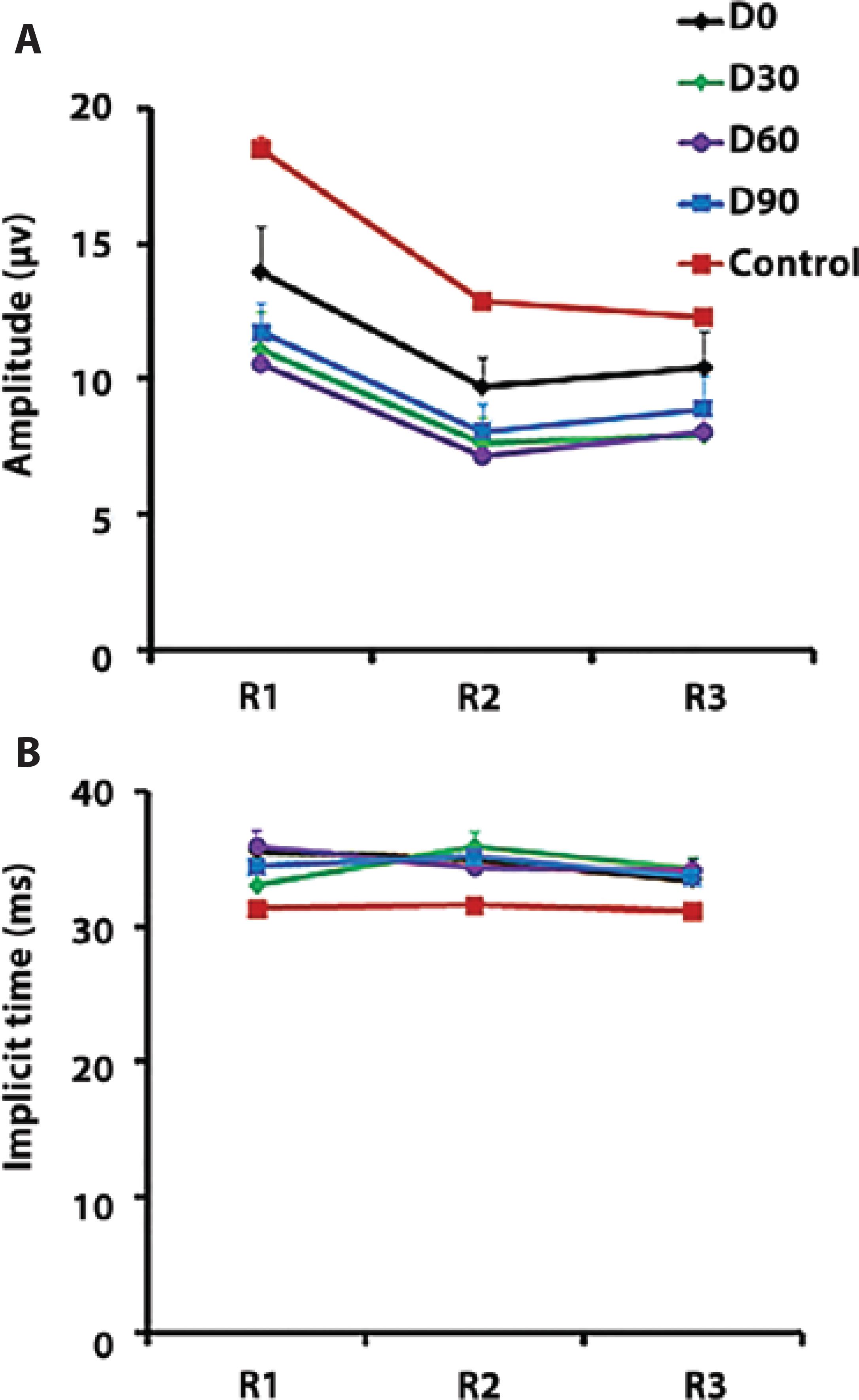
Figure 4 (A) Amplitude and (B) implicit time of the P1 component of the multifocal electroretinogram (mfERG).
In R1, P1 implicit time at D0 and D60 was significantly delayed in patients compared with that in the control group (P<0.05, one-way ANOVA, Tukey’s post-hoc test; Figure 4 B). In R2, P1 implicit time was delayed at D30, D60, and D90 in patients compared with that in controls (P<0.01, one-way ANOVA, Tukey’s post-hoc test; Figure 4 B). In R3, P1 implicit time at D30 and D60 was significantly delayed in patients compared with that in controls (P<0.05, one-way ANOVA, Tukey’s post-hoc test; Figure 4 B).
Tables 1 and 2 show the mean values of the amplitude of mfERG components along the therapy.
Table 1 mfERG amplitude and implicit time of the N1 component for three retinal areas at different days of therapy. Averaged values for controls are shown for comparison
R1 amplitude (μv) | R2 amplitude (μv) | R3 amplitude (μv) | |
---|---|---|---|
GT-D0 | -11.43 ± 0.99** | -6.24 ± 0.43* | -5.84 ± 0.51* |
GT-D30 | -8.09 ± 0.61* | -4.41 ± 0.37* | -4.71 ± 0.50* |
GT-D60 | -8.54 ± 0.79** | -4.41 ± 0.37* | -4.49 ± 0.43* |
GT-D90 | -9.88 ± 0.99** | -5.18 ± 0.48* | -4.67 ± 0.46* |
GC | -13.87 ± 0.95 | -8.46 ± 0.58* | -7.26 ± 0.50* |
R1 implicit time (ms) | R2 implicit time (ms) | R3 implicit time (ms) | |
GT-D0 | 17.79 ± 0.69 | 16.80 ± 0.54 | 15.47 ± 0.21* |
GT-D30 | 15.23 ± 1.19 | 17.76 ± 0.91 | 16.46 ± 0.34* |
GT-D60 | 14.02 ± 0.92 | 16.18 ± 0.93 | 16.04 ± 0.41* |
GT-D90 | 17.06 ± 0.46 | 16.81 ± 0.61 | 15.83 ± 0.23* |
GC | 15.74 ± 0.48 | 14.94 ± 0.23 | 15.16 ± 0.21* |
GT= group of patients receiving therapy; GC= group of control subjects; D0= day of the first injection; D30= 30th day after therapy initiation; D60= 60th day after therapy initiation; D90= 90th day after therapy initiation;
*= P<0.01;
**= P<0.05
Table 2 mfERG amplitude and implicit time of the P1 component for three retinal areas at different days of therapy. Averaged values for controls were also shown for comparison
R1 amplitude (μv) | R2 amplitude (μv) | R3 amplitude (μv) | |
---|---|---|---|
GT-D0 | 14.24 ± 1.61* | 10.26 ± 1.06* | 11.10 ± 1.27 |
GT-D30 | 11.57 ± 1.33* | 7.89 ± 0.93* | 8.29 ± 1.08 |
GT-D60 | 11.21 ± 0.95* | 7.64 ± 0.79* | 8.50 ± 0.90 |
GT-D90 | 12.39 ± 1.10* | 8.39 ± 0.90* | 9.14 ± 1.15 |
GC | 18.57 ± 1.25* | 13.26 ± 0.89* | 12.79 ± 0.94 |
R1 implicit time (ms) | R2 implicit time (ms) | R3 implicit time (ms) | |
GT-D0 | 35.36 ± 0.76** | 34.73 ± 0.71* | 33.38 ± 0.51* |
GT-D30 | 32.94 ± 0.98** | 35.65 ± 0.97* | 34.19 ± 0.79* |
GT-D60 | 35.67 ± 1.14** | 34.21 ± 0.54* | 32.62 ± 0.33* |
GT-D90 | 34.31 ± 1.07** | 34.91 ± 0.68* | 33.55 ± 0.63* |
GC | 31.44 ± 0.32** | 31.61 ± 0.22* | 31.20 ± 0.15 |
GT= group of patients receiving therapy; GC= group of control subjects; D0= day of the first injection; D30= 30th day after therapy initiation; D60= 60th day after therapy initiation; D90= 90th day after therapy initiation;
*= P<0.001;
**= P<0.001.
DISCUSSION
In a multicenter study (the MARINA Study), Boyer and colleagues found improved visual acuity in the ranibizumab-treated group(12), a result corroborated by another multicenter study(13). Most multicenter studies evaluated only patients with good visual acuity, and a few studies evaluated the response of anti-angiogenic therapy in patients with advanced AMD. Despite subjective reports of improved perception in the central scotoma, visual acuity remained altered, with no significant difference before and after treatment. However, a significant reduction in retinal thickness was observed by measuring total macular volume before and after treatment. The results of this work showed a stabilization of visual acuity in patients before and after treatment, but the patients’ visual acuity was different from that observed in previous studies.
There was a significant reduction in retinal thickness after the first month of treatment compared with other days of examination, which is in agreement with other studies(14,15). Keane et al. used OCT to study 95 patients with neovascular AMD after intravitreal injections of ranibizumab(14). The total subretinal fluid (SRF) volume reached its lowest level by 1 month after the injection (P<0.001). Karagiannis et al. evaluated 34 patients treated with monthly injections of intravitreal bevacizumab for six months followed by monthly injections of ranibizumab for 12 months(15). In these patients, OCT showed a decrease in retinal thickness (P=0.033) after bevacizumab, and this reduction improved after changing to ranibizumab (P=0.09).
Some authors suggested that patient history and age should be considered before analyzing mfERG since these factors may alter the retinal response. Importantly, mfERG responses became smaller and delayed with advancing age(16). The responses mediated by retinal cones occurring before retinal damage became observable by ophthalmoscopy(17). Some studies suggested that the implicit time of the photopic mfERG responses was more sensitive for detecting areas with retinal dysfunction than response density(18).
We estimated the amplitude and implicit time mean values for N1 and P1 mfERG components as a function of the time after ranibizumab injections. We focused on the temporal evolution of these parameters along the therapy. In this study, constant factors that could change these parameters, such as the hexagon areas, were not considered when estimating amplitude density. Previous studies revealed a delay in N1, P1, and the second negative component (N2) in 16%, 23%, and 31%, respectively, but response density decreased by only 4.2% with the stimulated area(19). A study evaluating only two patients with exudative AMD demonstrated a decrease in mfERG responses but no correlation between lesion size and response density or visual acuity(20). Another study that evaluated nine patients with AMD found no changes in ffERG after injecting bevacizumab (Avas-tin), but some improvement was detected in the mfERG responses, suggesting that the drug improved macular function as indicated by a better mfERG response and was not toxic to the retina once there were no ffERG changes(21). Feigl et al.(4) evaluated the mfERG response in three patients before, during, and after ranibizumab treatment. They divided the stimulus in two concentric rings (R1 and R2) and found no significant differences in N1 and P1 amplitudes for R1 or R2 prior to treatment in patients compared with those in controls. After the third treatment, N1 and P1 amplitudes in R1 and R2 significantly decreased compared with those in controls. The implicit time of N1 and P1 in R1 and R2 did not change after treatment, but it was significantly delayed in two out of three patients when compared with those in controls. The authors argued that the mfERG represented AMD neovascularization (mfERG evaluated retinal function in up to 25° of the visual angle and thus represents the area affected by the neovascularization membrane), whereas visual acuity reflected the function of the foveal area, a region <1° of the visual angle. They suggested that the adverse effects of anti-VEGF in affected retinal areas were larger than the area clinically affected by AMD(4).
The role of VEGF in choroidal neovascularization is well established(22). Inflammation and focal retinal ischemia induce VEGF production, leading to the proliferation of neovascular membranes from the choriocapillaris(23). Ranibizumab was demonstrated as acting as anti-ocular VEGF; it neutralizes VEGF but whether it has an adverse effect on the healthy peripheral retina is not clear(24). Some studies suggest that VEGF inhibition may cause retinal ischemia(25).
The present work suggests a stabilization of visual acuity and reduction of edema after treatment, which is in agreement with other studies(26). Costa and colleagues suggested that the edema reduction alone could result in an improvement and/or stabilization of visual acuity(27). However, conflicting mfERG data were reported in other studies and suggested that AMD interrupted delicate intraretinal mechanisms. The progression of the disease associated or not with the adverse effects of anti-VEGF could interfere with neuroretinal functions(28).
We observed a reduction in the electrical activity of the central macular region (R1) after the initiation of treatment in patients undergoing anti-angiogenic therapy with ranibizumab. As AMD affected mainly the central region, changes were expected in R1, which had the largest concentration of retinal neurons affected by the disease and was the place where the drug’s anti-angiogenic effect was expectedly higher(4). Some experimental studies suggested a possible toxic effect of high-dose intravitreal VEGF inhibitors on retinal neurons(29,30). The lower retinal electrical activity observed immediately after treatment raised the possibility of a deleterious effect of the medication on macular function, which fortunately recovered over time. Perhaps the preferential occurrence of macular lesions in advanced AMD facilitated a very large increase in the levels of anti-angiogenic factors in the central retina, temporarily causing this deleterious effect.
The present study showed a reduction of retinal thickness due to a reduction of edema and tissue fibrosis. The mfERG findings showed that the amplitude of mfERG components decreased and that the implicit time of these components increased, suggesting a worsening of retinal activity. The non-significant reduction in visual acuity indicated that retinal activity impairment did not affect it. Along the therapy, we found an improvement of the mfERG response. More controlled studies will be necessary for more conclusive results of the effects of ranibizumab on AMD.