INTRODUCTION
Age-related macular degeneration (AMD) is the most common cause of irreversible blindness among individuals aged over 65 in developed countries1,2. The global prevalence of AMD is reportedly 8.69% in people aged 45-85 years, and the number of patients with AMD is projected to increase to approximately 196 million in 2020 and 288 million in 20403. Globally, the costs directly associated with AMD are estimated to be $255 billion4. Furthermore, the proportion of people aged over 60 years is increasing rapidly in almost every country5, resulting in a dramatic increase in the global societal and economic burden of AMD.
The pathogenesis of AMD remains poorly understood. However, there is a general consensus that retinal damage occurs with aging as a result of factors such as oxidative damage, parainflammatory dysregulation, and vascular sclerosis6,7. Moreover, the free-radical theory of aging proposes that reactive oxygen intermediates (ROIs), such as free radicals, hydrogen peroxide, and singlet oxygen, are responsible for the development of age-related disorders8. If the free-radical theory applies, antioxidant/oxidant homeostasis may be disrupted in patients with AMD.
The organic sulfur derivative thiols are one of the most important components of the antioxidant-defense system9. They react with the electrophilic groups of ROIs, forming disulfide bonds that facilitate reversible thiol disulfide-exchange reactions10. In this study, we evaluated dynamic thiol/disulfide homeostasis (TDH) in plasma samples. We also measured malondialdehyde (MDA) levels and catalase (CAT) activity.
METHODS
Thirty-two patients with exudative AMD and 38 age- and sex-matched healthy controls were enrolled at a university hospital. All patients provided written informed consent before enrollment. All procedures conformed to the tenets of the Declaration of Helsinki. The study was approved by the Ethics Committee of the Diskapi Yildirim Beyazit Training and Research Hospital, Ankara, Turkey.
Detailed ophthalmologic examinations were performed, including visual acuity testing, slit-lamp biomicroscopy, retinoscopy, intrao cular pressure measurement via Goldmann applanation tonometry, optical coherence tomography, and fluorescein angiography. Patients included in the study had either choroidal neovascularisation or disciform scars. Participants with any systemic disease that could affect TDH, such as a chronic inflammatory disease, rheumatologic disease, diabetes mellitus, hypertension, cardiovascular disease, or malignancy, as well as smokers, alcoholics, patients with a body mass index greater than 30 kg/m2, and anyone taking antioxidant supplements, were excluded from the study.
All tests were conducted on plasma samples. Venous blood samples were collected in tubes containing ethylenediaminete traacetic acid and centrifuged at 1500× g for 10 min. Plasma samples were frozen and stored at -80°C until analysis. Native thiol, total thiol, and disulfide levels and TDH status were determined using a novel, automated assay developed by Erel and Neselioglu11. Briefly, the assay reduced dynamic disulfide (-S-S-) bonds to form free functional thiol groups (-SH) using sodium borohydride. Then, the unreacted sodium borohydride was removed with formaldehyde. The thiol groups (reduced and native) were detected upon reaction with 5,5'-dithiobis-(2-nitrobenzoic) acid. The number of disulfide bonds was calculated as half the difference between the total and native levels of thiol. Following the measurement of native thiol and disulfide concentrations in the pretreated samples, the dynamic TDH was determined.
CAT activity was determined using the methods described by Aebi12. The rate of decomposition of the H2O2 substrate by the CAT enzyme was measured spectrophotometrically at 240 nm. MDA levels were detected using the thiobarbituric acid method, and the formation of a pink-colored complex was measured spectrophotometrically at 532 nm13.
Statistical analysis
Statistical analysis was performed using SPSS software (Version 22.0; IBM Corp., Armonk, NY, USA). The Kolmogorov-Smirnov test was used to determine the normality of data distribution. Because the distribution of everything except CAT activity was normal, the Student's t-test for independent means was used to explore the significance of the differences between measurements in the two study groups. The Mann-Whitney U-test was used to compare the differences in CAT activity. p values <0.05 were considered to indicate statistical significance.
RESULTS
Table 1 presents the demographic details of the patient and control groups. There were no significant differences between the patient and control groups in age (p=0.148) or sex (p=0.079). Native thiol, total thiol, and disulfide levels, TDH status, MDA levels, and CAT activities are also given in table 1. Native thiol levels (272.02 ± 52.41 µmol/L) were significantly lower (p=0.004) in patients with AMD than in healthy individuals (307.82 ± 47.18 µmol/l; Figure 1). However, no significant differences were evident in total thiol levels (Figure 1). Disulfide levels were significantly higher (p<0.001) in patients with AMD (21.64 ± 5.59 µmol/l) than in controls (14.48 ± 5.37 µmol/L; Figure 2 A). Dynamic TDH was also significantly lower (p<0.001) in patients with AMD (13.41 ± 4.3 µmol/L) than in controls (25.41 ± 14.52 µmol/L; Figure 2 B). No significant differences were evident in MDA levels (Figure 2 C). Mean CAT activity was significantly higher (p=0.043) in patients with AMD (0.035 k/ml) than in controls (0.018 k/ml; Figure 2 D).
Table 1 Demographic and biochemical data of the AMD patients and control group
AMD patients (n=32) (mean ± SD) (minimum-maximum) | Controls (n=38) (mean ± SD) (minimum-maximum) | p* | |
---|---|---|---|
Sex (female/male) | 12/20 | 21/17 | <0.079 |
Age | 73.81 ± 6.3 (59-84) | 71.66 ± 5.9 (57-85) | <0.148 |
Native thiol, µmol/L | 272.02 ± 52.41 (140.4-345.9) | 307.82 ± 47.18 (167.6-418.8) | <0.004 |
Native thiol/disulfide, % | 13.41 ± 4.3 (5.83-23) | 25.41 ± 14.53 (11.34-79.54) | <0.001 |
Disulfide, µmol/L | 21.64 ± 5.59 (12.35-35.1) | 14.48 ± 5.38 (5-26.25) | <0.001 |
Total thiol, µmol/L | 315.3 ± 52.46 (188.6-391.5) | 336.78 ± 49.31 (186.9-464.5) | <0.082 |
MDA, nmol/ml | 29.36 ± 8.34 (14.12-58.24) | 33.29 ± 19.09 (23.24-144.12) | <0.284 |
CAT, k/ml | 0.035 ± 0.046 (0.002-0.194) | 0.018 ± 0.049 (0.003-0.49) | <0.043 |
*comparisons with control eyes using the Student's t-test. p<0.05 was considered statistically significant.
The χ2 test was used to compare sex distribution. AMD= age-related macular degeneration; SD= standard deviation= MDA; malondialdehyde; CAT= catalase.
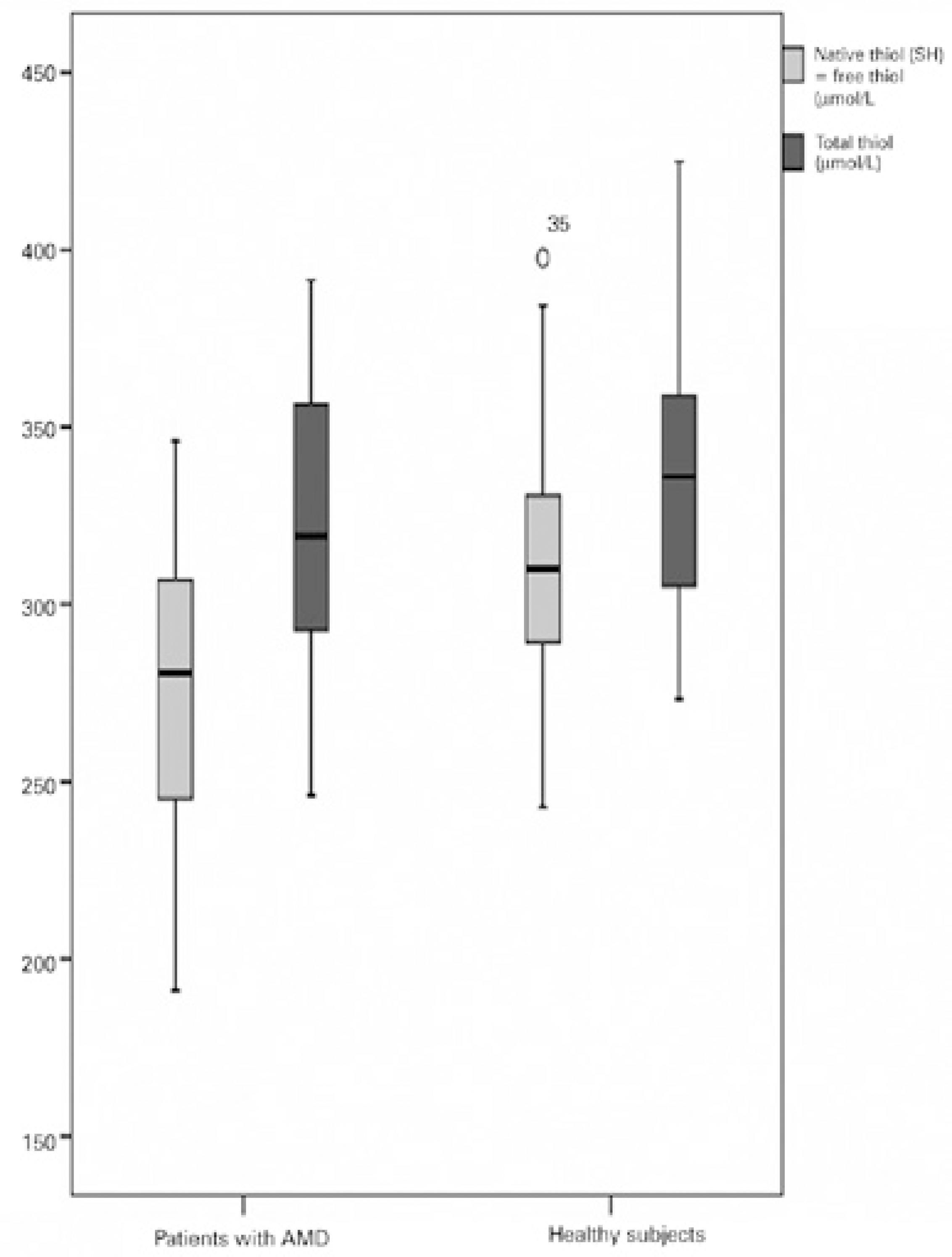
Figure 1 Comparison of native and total thiol levels in the plasma of patients with age-related macular degeneration versus healthy controls.
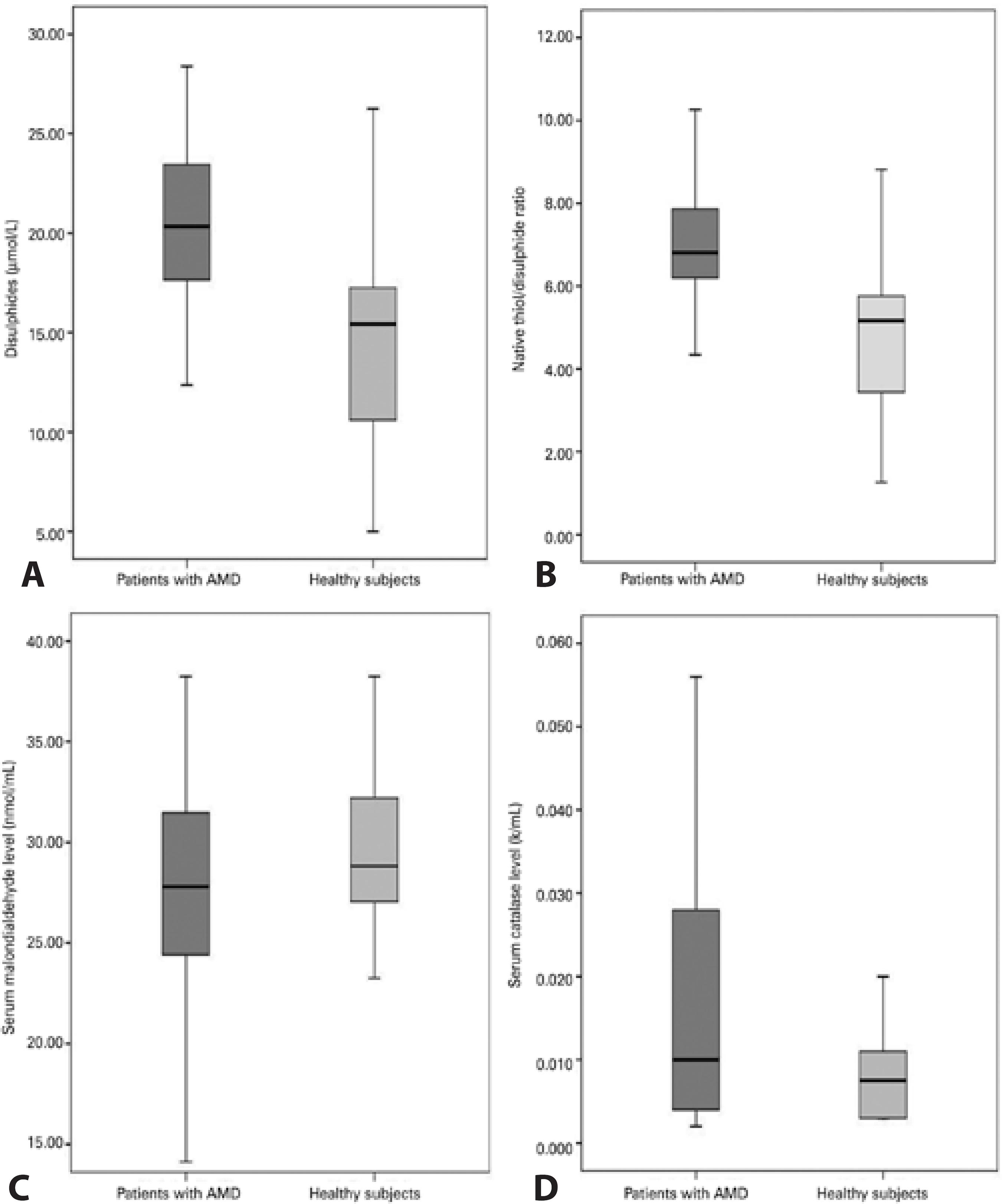
Figure 2 Comparative levels of oxidative stress markers in the blood of AMD patients and controls. A) Disulfide levels in the plasma of patients with age-related macular degeneration (AMD) and healthy controls. B) Native thiol/disulfide homeostasis in patients with AMD and healthy controls. C) Plasma levels of malondialdehyde in patients with AMD and healthy controls. D) Mean catalase activity in the plasma of patients with AMD and healthy controls.
DISCUSSION
We used a novel and automated assay to determine TDH. Native thiol concentrations and thiol/disulfide ratios were significantly lower, and disulfide levels were significantly higher in patients with AMD than in controls. To the best of our knowledge, this is the second study to investigate dynamic TDH as a novel marker of oxidative stress in patients with AMD and compare the results with a control group.
Although the pathogenesis and etiology of AMD remain poorly understood, there is considerable evidence that oxidative stress plays a role in the onset and progression of the disease. The first di rect evidence of a causal relationship between oxidative stress and aging and age-related disorders was reported in a study involving transgenic flies (Drosophila melanogaster): flies overexpressing genes for antioxidant enzymes (copper-zinc superoxide dismutase and CAT) exhibited a longer lifespan and a delayed loss of physical performance14.
Several studies that evaluated total thiol concentration in plasma samples reported significantly reduced thiol levels in patients with exudative AMD18-20. To the best of our knowledge, the only study to investigate TDH to date reported that patients with advanced AMD exhibited significantly lower levels of TDH than healthy controls (20.3 ± 1.2 vs. 29.5 ± 3.1, p=0.005)20. In this study, dynamic TDH was also significantly lower (p<0.001) in patients with AMD (13.41 ± 4.3 µmol/L) than in controls (25.41 ± 14.52 µmol/L). There were no statistically significant differences in total thiol levels, but the native (free) thiol concentration (272.02 ± 52.41 µmol/L) was significantly lower (p=0.004) in patients with AMD than in healthy individuals (307.82 ± 47.18 µmol/L). This supports the conclusions of previous studies that rather than the total thiol concentration, the thiol/disulfide balance has a fundamental role in protection against oxidative stress21,22.
CAT is an iron-dependent antioxidant enzyme that scavenges H2O223. Although this enzyme may play an important role in antioxidant-defense in the retina, published reports regarding the relationship between systemic and retinal CAT activity and AMD are contradictory. Orr and Sohal14 reported significantly reduced CAT activity in the retinal pigment epithelium (RPE) of eyes with AMD. Tate et al.24 observed increased CAT activity in the RPE in response to a challenge with exogenous H2O2, suggesting that phagocytosis of the outer segments of the rods near the RPE is a response to oxidative stress that probably produces H2O2, which is believed to act as an intracellular signal that induces CAT activity. In this study, the mean CAT activity was significantly higher (p=0.043) in patients with AMD (0.035 k/ml) than in controls (0.018 k/ml). We believe that CAT activity, like other components of the antioxidant-defense system, is dependent on many factors, such as genetic variation, nutrition, and disorders, which may have influenced the systemic or retinal CAT activities observed in previous studies. However, as discussed in a previous study24, our findings suggest a relationship between increased systemic CAT activity and AMD.
MDA is a common lipid peroxidation product and reliable marker of oxidative stress25. Drusen, the hallmark of AMD, contains MDA, which can damage the RPE26. Studies that evaluated MDA concentrations in plasma samples reported significantly increased levels of MDA in patients with exudative AMD27,28. In this study, no significant differences were observed in MDA levels. As addressed by our exclusion criteria, many factors can affect the antioxidant-defense system. However, other confounding factors that were not taken into consideration, such as genetic structure, nutrition, and disorders such as atherosclerosis, may explain the discrepancies between the results of different studies.
There were several limitations to this study. Because of the small sample size, our results are difficult to translate to all patients with AMD. Detection of TDH in vitreous samples can be useful for assessing oxidative stress in the retina, but this was not performed in this study. Aside from age-related diseases including AMD, many factors such as genes, environment, and diet may alter TDH and an tioxidant status. This situation may have resulted in a bias in participant enrollment.
In conclusion, this is the second study to examine TDH in patients with AMD. The antioxidant/oxidant balance of dynamic TDH shifts to the oxidative side in patients with AMD. These findings support previously reported evidence of a causal relationship between oxidative stress and AMD. Oxidative stress markers may be disease specific, and not all markers are disturbed in AMD. Further studies involving larger patient populations are warranted to determine the relationships between systemic markers of oxidative stress and AMD.