Introduction
Ischemia-reperfusion (I/R) injury occurs because of temporary disruption and subsequent improvement of the blood flow. This type of injury may become serious because significant amounts of reactive oxygen species (ROS), which are very toxic to cells, are produced as a result of tissue reoxygenation. Both direct and indirect mechanisms may be involved in ROS-induced cellular toxicity. In the direct mechanism, because both mitocondria and DNA are surrounded by membranes, ROS may lead to disruption of mitocondrial function and alterations in the structure of DNA, as a result of peroxidation of polyunsaturated fatty acids present in these membranes(1). In the indirect mechanism, ROS induce hypersecrection of excitatory amino acids such as glutamate and aspartate(2). Hypersecrection of excitatory amino acids leads to an influx of excessive free calcium by stimulation of the N-methyl-D-aspartate (NMDA) receptor-operated channels, and increased levels of intracellular free calcium may subsequently trigger apoptosis of neuronal cells by a process called excitotoxicity(2,3). Neurotrophin, deprivation, glial activation, ischemia, glutamate excitotoxicity, and oxidative stress lead to induction of apoptotic cell death of retinal ganglion cells(3).
Retinal cell death is mostly due to apoptosis rather than necrosis, and this provides an important advantage to the retina because apoptosis does not result in an extreme inflammatory response; thus, limiting cell loss(4). However, in the event of excessive or deficient apoptosis, such as in I/R injury, increased loss of retinal neurons may lead to visual impairment if the macula is affected(5,6). I/R injury is the main pathogenetic mechanism underlying several clinically important diseases such as myocardial infarction, stroke, spinal injury, and shock, as well as some sight-threatening disorders such as diabetic retinopathy, retinal vascular occlusion, and glaucoma(7). Thus, there may be a potential benefit from the use of medications, vitamins, and substances possessing both antioxidative and antiapoptotic effect as adjuvants in the treatment of eye diseases associated with I/R injury.
Flavonoids are plant-derived substances possessing several antioxidative properties. Among the flavonoids, quercetin is known as a powerful antioxidant and free radical-scavenging substance(8). Furthermore, quercetin also posseses antiapoptotic effects(9). Some studies have evaluated the antiapoptotic effect of quercetin; however, little data is available regarding the antiapoptotic effect of quercetin on retinal I/R injury(10,11).
In the present study, we aimed to evaluate whether quercetin decreases apoptotic cell loss in a rat retinal I/R injury model. Because the intensity of apoptosis occuring in the different layers of the retina may differ because of differences in the susceptibility of the retinal layers to I/R injury, we separately evaluated the antiapoptotic effect of quercetin on both the inner and outer nuclear layers of the retina.
METHODS
Twenty-four male Winstar Albino rats weighing 250-300 g, which were obtained from Saki Yenilli Research Animal Laboratory, were included in the study. After obtaining approval from Canakkale Onsekiz Mart University Ethics Committee of Experimental Animals, the rats were housed in cages with free access to standard food and drinking water, and they were maintained under controlled conditions, including a 12-h light/dark cycle (08:00-20:00, light; 20:00-08:00, dark), an ambient temperature of 23-25°C, and a humidity in the range of 55%-60% in the Canakkale Onsekiz Mart University Laboratory of Animal Research Center.
All operations were performed under aseptic conditions. For anesthetizing the rats, a single dose of 80 mg/kg ketamine (Ketasol, Richter Pharma Ag, Wels, Austria) and 5 mg/kg xylazine (Rompun, Bayer, İstanbul) was intraperitonally injected. To maintain body temperature within the physiological range, the rats were moved to special containers to ensure sufficient covering of their bodies. After instilling one or two drops of 0.5% proparakain HCl (Alcaine; Alcon, USA) for corneal analgesia, 10% povidone iodine was administered into the conjonctival sac to ensure desinfection of the ocular surface.
Twenty-four rats were divided into four groups of six according to weight and were used as control, ischemic, solvent, and quercetin treatment groups, respectively. In the control group, the right anterior chamber of each animal was penetrated without drug administration.
In the ischemic group, the I/R injury model was developed using the method previously described by Buchi et al.(12). According to this method, to establish retinal ischemia, the right anterior chamber was cannulated with a 30-gauge needle attached to an infusion set, and its reservoir was placed approximately 200 cm above the animal. After opening the reservoir of the infusion set containing physiological saline, the intraocular pressure (IOP) was raised above 50 mmHg, until whitening of the iris and loss of the red reflex of the fundus were observed, which are signs of retinal ischemia. All procedures and clinical examinations in the present study were performed using a portable biomicroscope (Shin Nippon, Japan). After 60 min of retinal ischemia, IOP was reverted to normal pressure by removing the infusion needle from the anterior chamber to allow the retinas to be reperfused. In the solvent group, after inducing retinal ischemia in the right eyes, di-methyl sulfoxide (DMSO) was intraperitoneally injected immediately before allowing the retinas to be reperfused. In the quercetin group, after inducing retinal ischemia in the right eyes, a dose of 20 mg/kg quercetin (Sigma-Aldrich Chemical Co., United Kingdom) dissolved in DMSO was intraperitoneally injected immediately before allowing the retinas to be reperfused. After 48 h, the right eye of each animal was enucleated to perform histopathological and immunohistochemical evaluations of the retina, and the experimental animals were sacrified.
Histopathological and immunohistochemical evaluations
Eye tissue samples, fixed in 10% buffered formaldehyde fixative solution and embedded in paraffin 48 h after acute retinal ischemia, were examined with hematoxylin and eosin (H&E) to evaluate histopathology. Sections that were 5 µm thick were obtained using a microtome (Leica, RM2245) and stained with H&E. Retinal thickness was calculated in three separate regions: peripheral (100-150 µm from the ora serrata), central (100-150 µm from the optic nerve), and midperipheral (halfway between the central and peripheral). Two representative sections were randomly selected from the same three positions for each eye, and their mean values were calculated(13). The sections were also incubated with polyclonal anti-Caspase-3 antibody (Abcam, ab4051; dilution 1:100) at room temperature for 90 min. Sections were washed three times with PBS and incubated with biotinylated secondary antibody (Ultra Vision Detection System-HRP kit, Thermo, Fremont, USA), and subsequently streptavidin peroxidase (Ultra Vision Detection System-HRP kit, Lab Vision, Fremont, USA) was added and sections were maintained at room temperature for 20 min.
Terminal deoxynucleotidyl transferase dUTP nick-end labeling (TUNEL) assay
Enucleated eyes were immersed in 10% formaldehyde fixative solution for 48 h at room temperature and embedded in paraffin; 5-µm sections were obtained, and the slides were air dried. Apoptotic cells were identified using terminal deoxynucleotidyl transferase dUTP nick-end labeling (TUNEL; Calbiochem, San Diego, CA, USA), as previously reported(14). Nuclear counterstaining was performed using 3,3’-diaminobenzidine (DAB), and sections were counterstained with hematoxylin.
Statistical analysis
Data were expressed as mean ± standard deviation (SE). The Bartlett test was used to determine whether the data were heterogeneous or homogeneous. Bonferroni multiple comparison test was applied to identify differences between means. Differences were considered statistically significant at probability (P) levels of <0.05.
RESULTS
The mean thicknesses of RGCL, IPL, OPL, INL, and ONL were 19 ± 2.7 µm, 60 ± 12.1 µm, 11 ± 3.0 µm, 27 ± 9.9 µm, and 55 ± 13.3 µm, respectively, in the control group; 8 ± 1.4 µm; 22 ± 6.5 µm, 7 ± 1.9 µm, 16 ± 6.4 µm, and 40 ± 8.7 µm in the ischemic group; 9 ± 0.9 µm, 22 ± 7.4 µm, 7.4 ± 1.6 µm, 17 ± 5.2, and 41 ± 10.2 µm in the solvent group; and 10 ± 3.8 µm, 25 ± 8.2 µm, 9 ± 2.4 µm, 21 ± 5.6 µm, and 50 ± 12.8 µm, respectively, in the quercetin group (Figures 1 and 2).
Values indicating the mean thicknesses of RGCL, IPL, and OPL measured in the quercetin group were statistically significantly greater those measured in the ischemic and the solvent group (P<0.05 and P<0.05 respectively). However, among all of the retinal layers, the reduction in the thicknesses of both ONL and INL was statistically significant, particularly in the quercetin group; ONL mean thickness in the quercetin and ischemic groups were 50 ± 12.8 µm and 40 ± 8.7 µm, respectively (P<0.05), and INL mean thickness in the quercetin and ischemic groups were 21 ± 5.6 µm and 16 ± 6.4 µm, respectively (P<0.05).
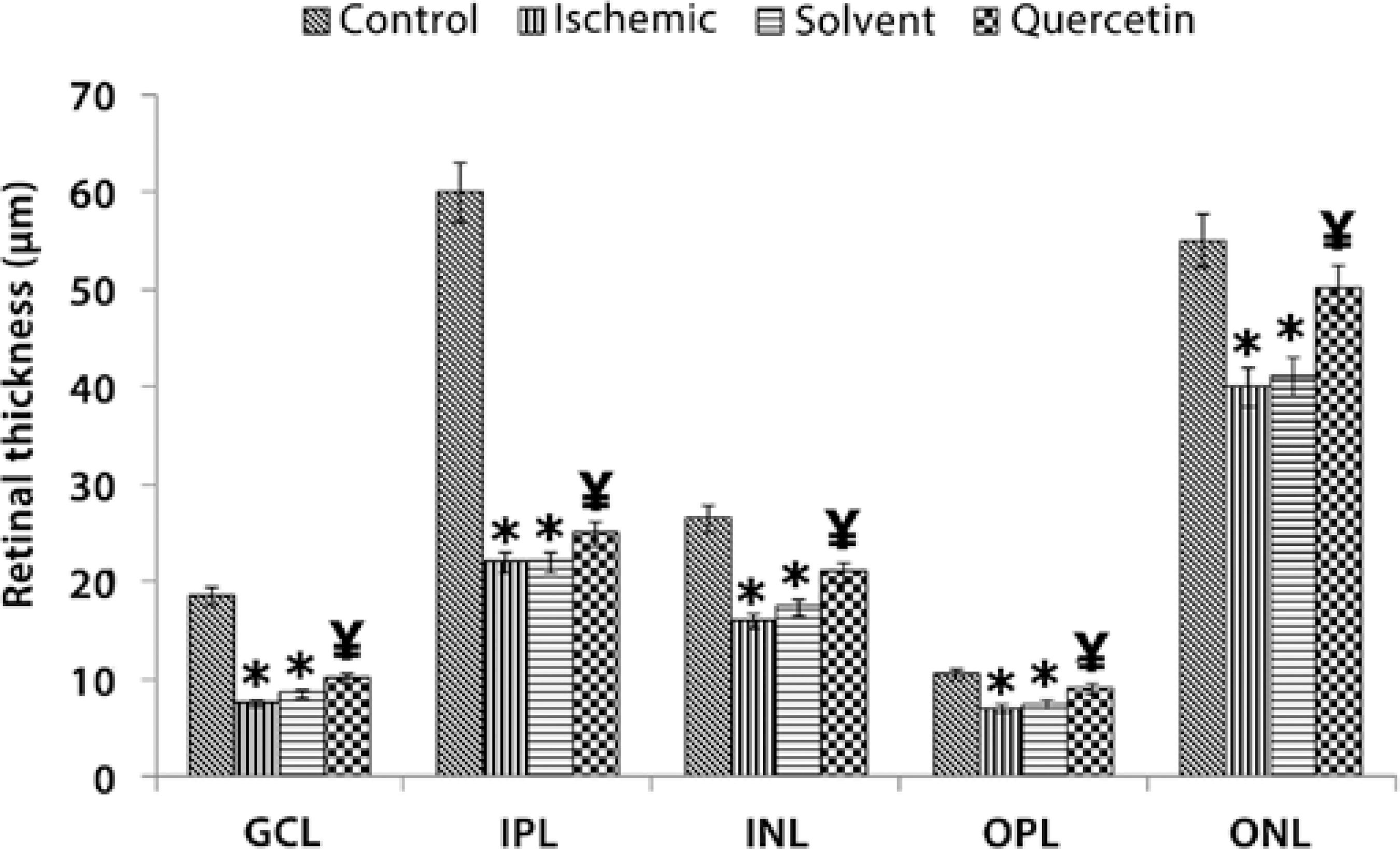
* P<0.05 compared with the control group.
¥ P<0.05 compared with the control, ischemic, and solvent groups.
Figure 1 The thicknesses of the retinal layers (µm) as measured in each group.
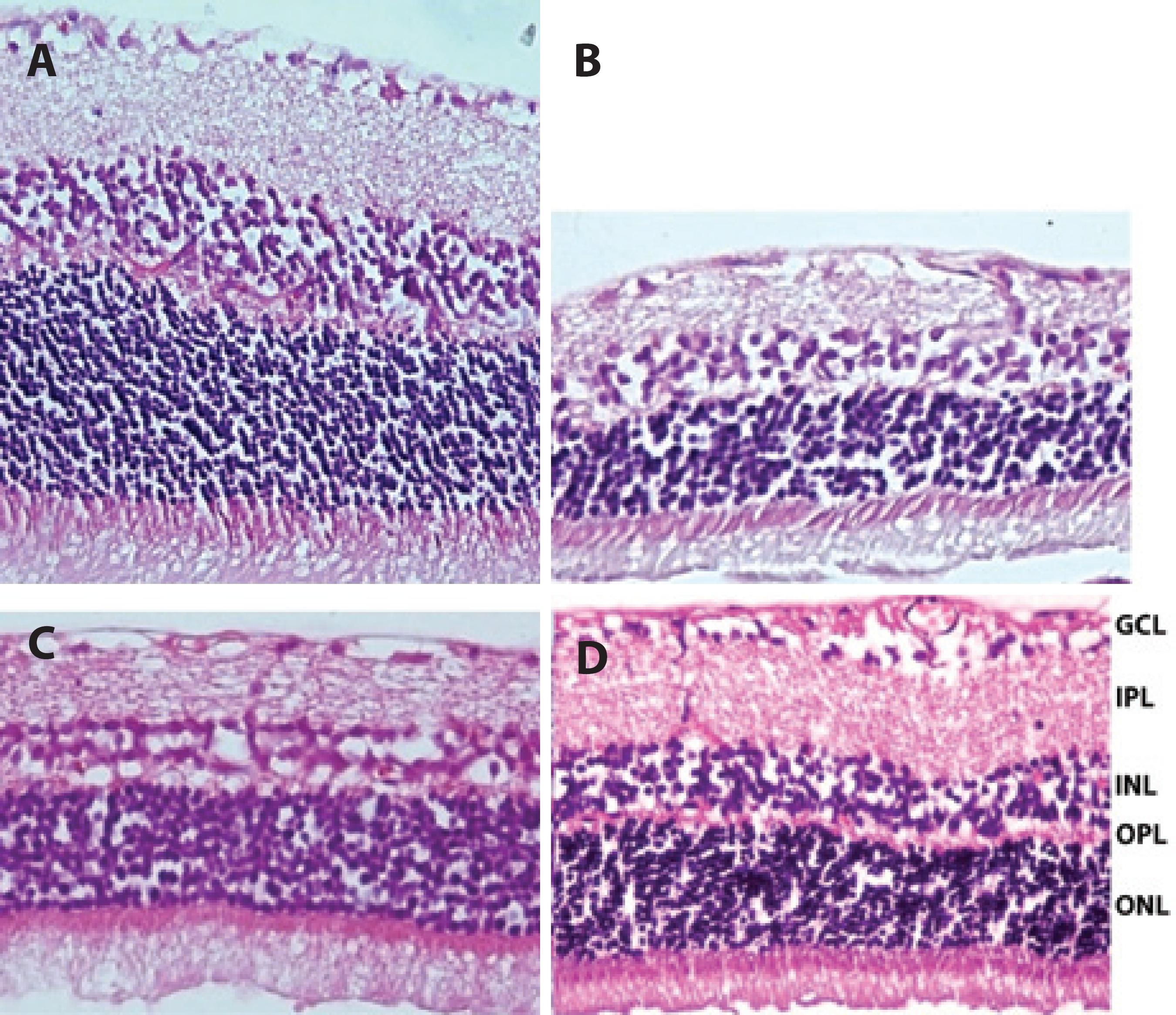
Figure 2 Representative photographs showing retinal thickness detected in the rat retina. A) Normal appearance of the retina (control group). B and C) Retinal thickness in the ischemic group and solvent group, respectively; in both of B and C, the thicknesses of the retina was found to be significantly reduced after ischemia. D) Retinal thickness in the quercetin group. Total retinal thickness in the quercetin group was significantly less reduced compared with the ischemic group at end of the experiment. H&E staining (×400).
The effect of quercetin on the number of TUNEL (+) cells in INL and ONL
The mean number of TUNEL (+) cells counted in INL was 10.88 ± 3.5/mm2, 476.8 ± 45.6/mm2, 336 ± 34.9/mm2, and 238.72 ± 251/mm2 in the control, ischemic, solvent, and quercetin groups, respectively. The mean number of TUNEL (+) cells in INL was significantly lower in the quercetin group than in the ischemic group (P<0.005). Moreover, the mean number of TUNEL (+) cells counted in ONL was 197.76 ± 18.4/mm2, 176 ± 16.8/mm2, and 71.68 ± 11.8/mm2 in the ischemic, solvent, and quercetin groups, respectively. The mean number of TUNEL (+) cells in ONL was also significantly lower in the quercetin group than in the ischemic group (P<0.05; Figures 3 and 4).
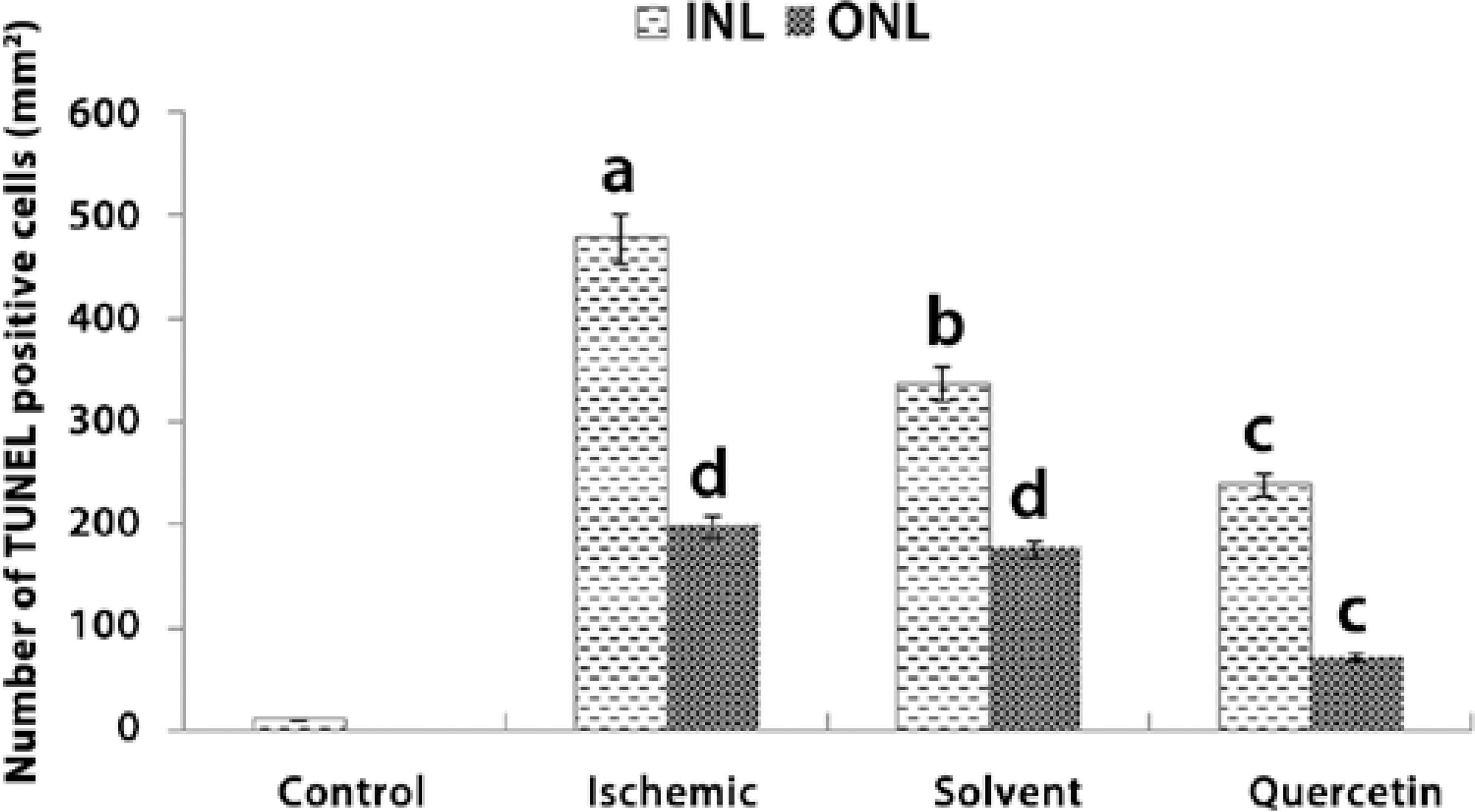
a= P<0.001 compared with the control group.
b= P<0.005 compared with the control and ischemic groups.
c= P<0.05 compared with the control, ischemic, and solvent groups.
d= P<0.05 compared with the control group.
Figure 3 Number of TUNEL (+) cells detected in the inner nuclear layer (INL) and outer nuclear layer (ONL) in each group.
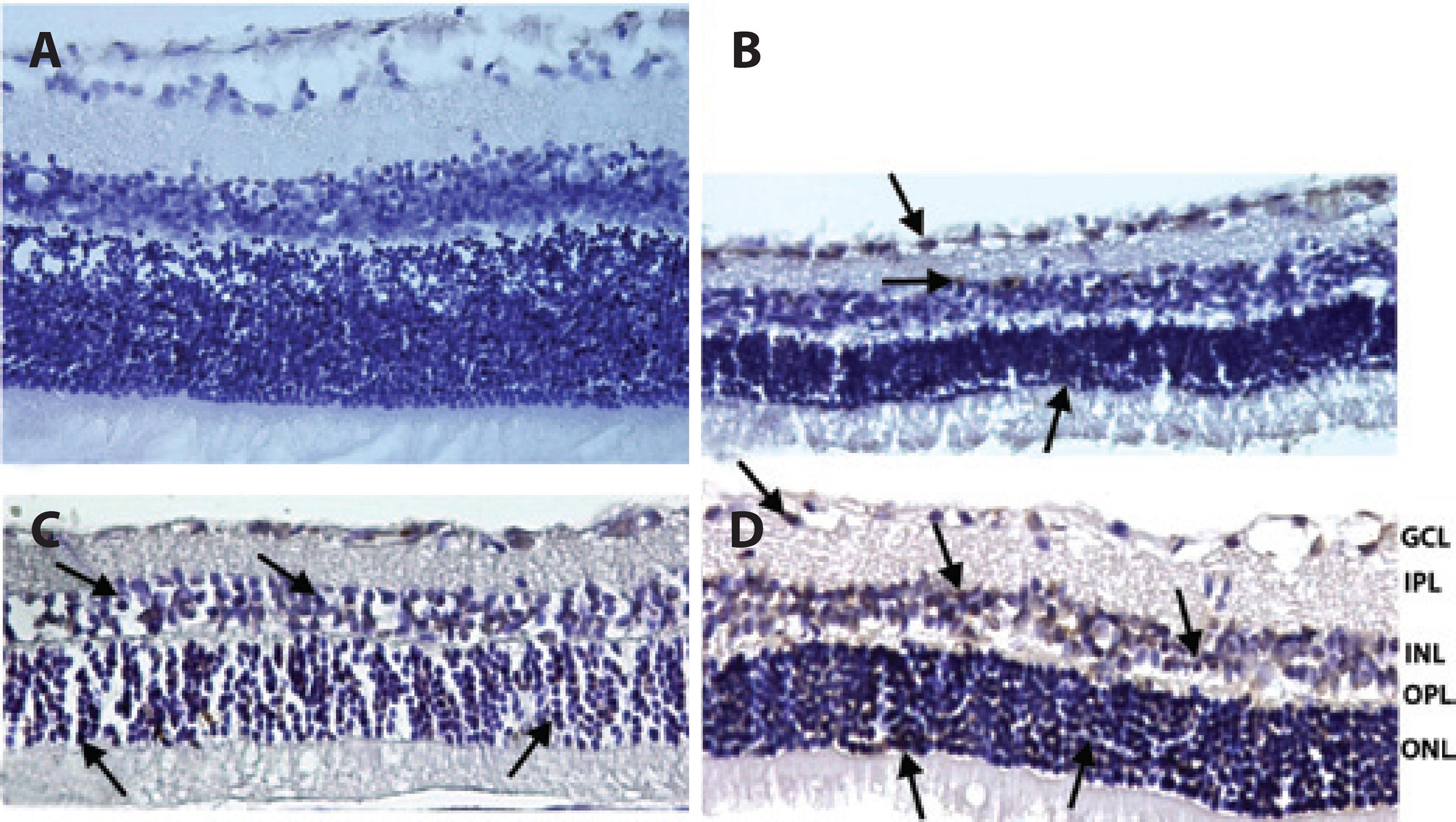
Figure 4 Representative photographs of TUNEL staining of rat retinas in control (A), ischemic (B), solvent (C), and quercetin (D) groups. The number of cells positive by TUNEL staining was increased in ischemic rats. However, treatment with quercetin markedly reduced retinal cell apoptosis. Arrows: TUNEL (+) cells. (×400).
Changes in the number of caspase-3 (+) cells in INL and ONL
The mean number of caspase-3 (+) cells detected in INL was 62.56 ± 0.2/mm2, 633.6 ± 38.7/mm2, 550 ± 34.2/mm2, and 342.4 ± 36.1/mm2 in the control, ischemic, solvent, and quercetin groups, respectively. Treatment with quercetin was found to significantly decrease the number of caspase-3 (+) cells in INL by a ratio of nearly 46% when compared with the ischemic group (P<0.05). Moreover, the mean number of caspase-3 (+) cells detected in ONL was 43.6 ± 9.4/mm2, 41.2 ± 6.7/mm2, and 29,7 ± 8.4/mm2 in the ischemic, solvent, and quercetin groups, respectively. Quercetin treatment was found to significantly decrease the number of caspase-3 (+) cells in ONL by a ratio of nearly 32% when compared with the ischemic group (P<0.05; Figures 5 and 6).
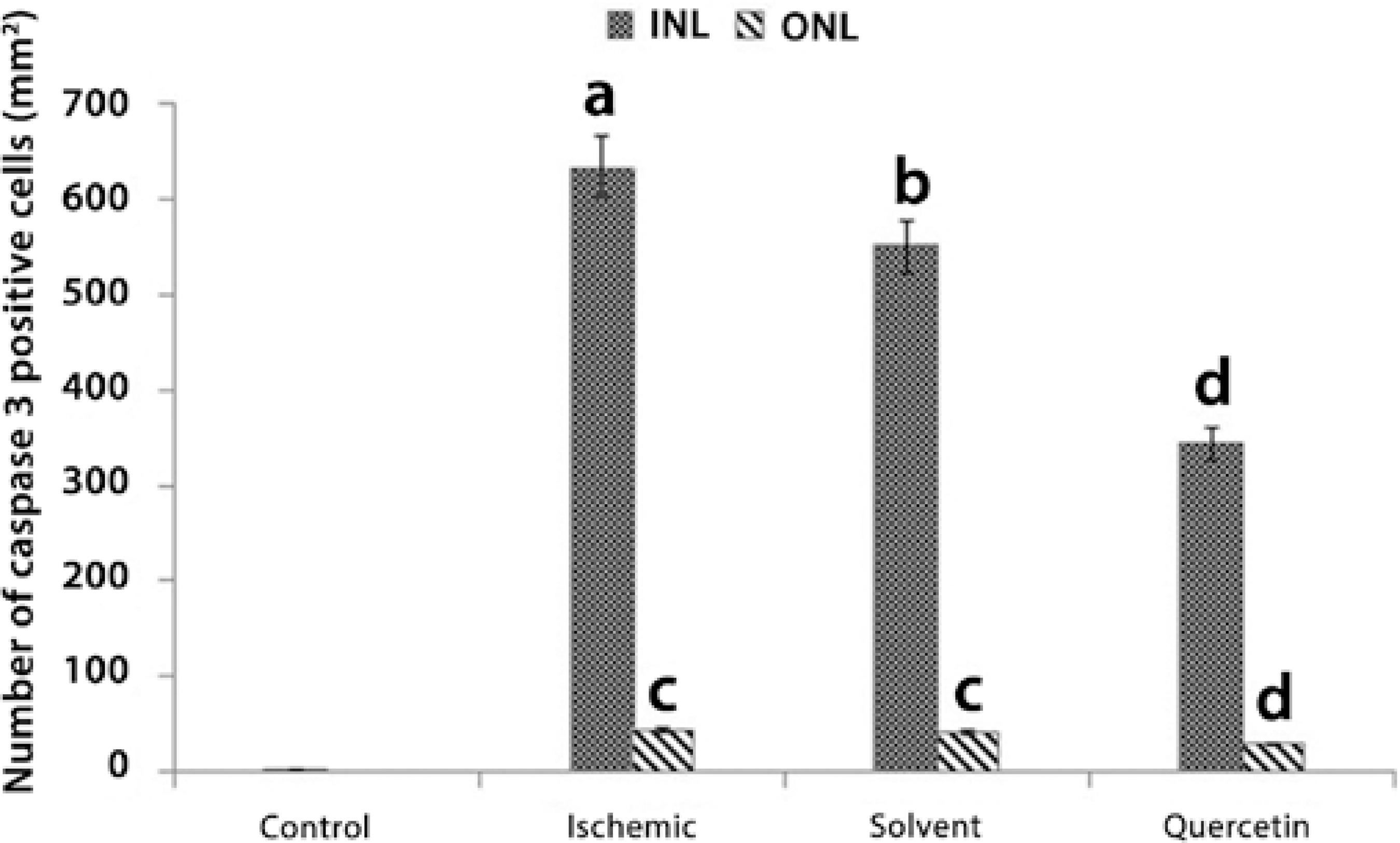
a= P<0.0001 compared with the control group
b= P<0.001 compared with the control and ischemic groups
c= P<0.005 compared with the control and quercetin groups
d= P<0.05 compared with the control, ischemic, and solvent groups
Figure 5 The number of caspase-3 (+) cells in the inner nuclear layer (INL) and outer nucler layer (ONL) in each group.
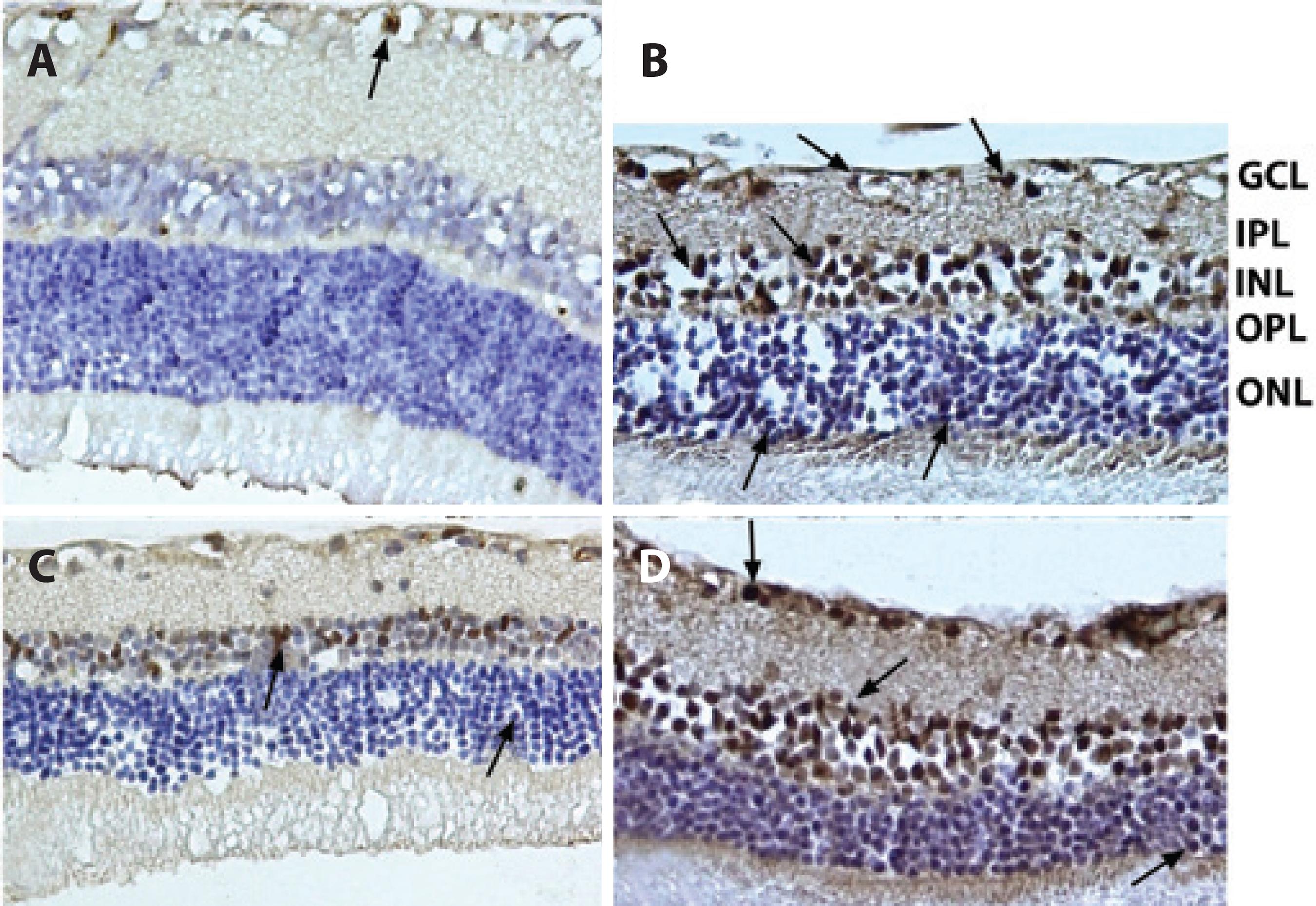
Figure 6 Representative photographs of caspase-3 (+) cells. A) Control group; B) Ischemic group; C) Solvent group, and D) Quercetin group. The number of caspase-3 (+) cells observed among the quercetin-treated retinal cells was significantly lower than that among the ischemic retinal cells. Arrows: Caspase-3 (+) cells. Immunoperoxidase, hematoxylin counterstain (×400).
DISCUSSION
The antioxidant, free radical-scavenging, and neuroprotective effects of quercetin is well known(15-17). Besides these important benefits of quercetin, it also has a protective effect against apoptotic cell damage. Some experimental studies have revealed the antiapoptotic effect of quercetin on I/R injury in various organs(18,19); however, we have not been able to find any experimental studies evaluating the benefit of quercetin in treatment of I/R injury of the retina.
Because apoptosis is the mechanism predominantly involved in retinal cell death, antiapoptotic drugs or substances are considered particularly beneficial in the treatment and prevention of retinal diseases that are associated with the formation of excessive apoptosis, such as I/R injury.
The exact mechanism of cell death due to retinal ischemia is incompletely known; however, it was previously demonstrated that in conditions of oxidative stress, retinal ganglion cells are damaged as a result of increased intracellular ROS and calcium influx(20). During I/R, overproduction of ROS leads to excessive apoptosis by inducing the secretion of glutamate and calcium influx(21-23). Apart from inducing apoptosis, increased intracellular calcium levels may also cause cellular damage by hindering mitocondrial functions, decreasing the level of intracellular adenosine triphosphate (ATP), inducing ROS production, and stimulating cellular proteases and nitric oxide synthase(24). Therefore, it is conceivable that the maintainance of intracellular free calcium levels at an optimal concentration may be critical for treating I/R injury.
Flavonoids, such as quercetin, contain a flavonol nucleus that is surrounded by functional groups that have the ability to maintain the intracellular free calcium concentration at low levels by influencing glutathione metabolism, particularly in conditions of excessive ROS production(25,26). Therefore, it may be reasonable to use quercetin in the treatment of I/R injury because of its beneficial biochemical properties.
A supressor effect of quercetin against the activities of caspase-3 and calpain, which are the representitive proteases in apoptotic and necrotic pathways, was demonstrated in purified primary rat retinal ganglion cell cultures and in human retinal pigment epithelium cell cultures(27,28). Moreover, an antiapoptotic effect of quercetin in terms of reducing caspase-3 activity has also been demonstrated in a myocardial I/R model(29). Because quercetin was found to induce cardioprotection in the myocardial I/R model, we thought that it may also be beneficial for retinal protection in the ocular I/R model; hence, we aimed to investigate this relationship in the present study.
To develop the ocular I/R model, we applied a widely used method, which involves increasing the intraocular pressure above the ocular perfusion pressure for nearly 60 min(5). In the histological examination of the retina, this procedure was found to cause retinal damage through different types of cell death mechanisms such as apoptosis, necrosis, and nonlysosomal vesiculate(5). Hughes demonstrated increased apoptosis and INL thinning in a tissue model of acute ischemic injury(30). In addition, a number of investigations have shown that a peak in apoptosis occurs 6 to 18 h after I/R, returning to control levels after 48 h(31,32). Therefore, in the present study, we administered quercetin immediately after the induction of ischemia but before the begining of reperfusion, i.e., before the peak of apoptosis. Forty-eight hours later, we found that the thickness of the retinal layers in the quercetin group was significantly less decreased than that in the ischemic and solvent groups.
Moreover, a significant decrease in the number of TUNEL (+) and caspase-3 (+) cells was detected in both INL and ONL in the quercetin group in comparison with the ischemic group. Because TUNEL (+) and caspase-3 (+) cells are important markers in the determination of apoptosis, obtaining a reduction in the number of such markers and a decrease in the thinning of retinal layers clearly demonstrates the antiapoptotic effect of quercetin on I/R-induced retinal injury. Although the decrease in the thinning of INL and ONL was found to be almost similar, the antiapoptotic effect of quercetin appeared much more evident in INL than in ONL. We believe that this disparity may arise from a difference in susceptibility of INL and ONL to ischemic damage. In a previous study, the intensity of apoptotic cell loss was found to be more significant in INL than in ONL in the earlier phases of I/R injury(33). Consistent with this finding, we also observed that the number of both TUNEL (+) and caspase-3 (+) cells was much higher in INL than in ONL.
In the present study, the reason for the significantly increased degree of apoptosis in INL may result from excitotoxicity, which is coupled to hypersecretion of excitatory amino acids such as glutamate. This idea is supported by the findings of both Massey et al. and Brandstätter et al. who showed that expression of glutamate receptors is confined to neurons of the ganglion cell layer and INL(34,35). This supports the hypothesis that quercetin may achieve its antiapoptotic effect depending on the intensity of the apoptosis, and it may be beneficial, particulary in the presense of increased apoptosis. On the other hand, because the number of both TUNEL (+)and caspase-3 cells was much lower in ONL in all the groups, it is speculated that the antiapoptotic effect of quercetin may not be prominent in ONL because of decereased induction of apoptosis. However, in an animal model of I/R injury, an increased rate of apoptosis in ONL was reported to be associated with extended postischemic survival time(36). In our study, upon evaluation of the early phases of I/R injury, we speculate that a significant decrease in the thickness of ONL in the ischemic group may also be due to a different cell death mechanism such as necrosis in addition to apoptosis. Therefore, the assessment of the intensity of apoptosis in ONL should be also elucidated in the later phases of the I/R injury model, to gain additional insight into the antiapoptotic effects of quercetin in all the layers of the retina.
Caspase-3 is an important marker of apoptosis, and it is capable of decreasing the thickness of retinal layers as a result of activating the proteolytic cascade(37). Hence in the current study, the possible mechanism of the effect of quercetin on change in retinal thickness over a relatively short time may arise from its inhibitory effect on caspase-3 cells. In a previous study, a dose of 20 mg/kg i.p. quercetin was found to be sufficient for achieving cerebral protection in a cerebral I/R injury model; we also used quercetin at a dose of 20 mg/kg i.p. in our retinal I/R injury model(38). Although, we could not assess the penetrance of quercetin into the retinal layers, we believe that when systemically used, quercetin may accumulate in the retinal layers at adequate concentrations during ischemic injury because of the possibly broken blood-retinal barrier. On the other hand, an antiapoptotic effect of quercetin was also previously demonstrated in the retinal layers of the streptozotocin-induced diabetic rats even after oral administration(39). This result may indicate that quercetin has a good oral bioavailability; therefore, if the therapeutic dose is adjusted for humans in future studies, oral use of quercetin may be beneficial in the treatment of various retinal diseases associated with I/R injury.
Toxicity studies of quercetin and electrophysiological tests of the retina could not be performed in the present study because of inadequate laboratory equipment; we view this as a major limitation of this study. Because we histologically examined the retinal tissues 48 h after I/R injury, the antiapoptotic effect of quercetin in the later periods of the injury could not be properly evaluated, and this is another major limitation of this study. However, we used an additional number of rats to create a control group. However, we believe that if we had used the left eyes of either the ischemic group or the solvent group as a control group, we would not have been able to perform accurate comparisons between the control group and the other experimental groups because of the influence of possibly increased inflammatory cytokines produced in the eye with I/R injury, as in the case of sympathetic ophthalmia.
CONCLUSION
To the best of our knowledge, this study is the first to demonstrate the neuroprotective effect of quercetin on apoptotic cell injury in a retinal I/R model. Because a significant antiapoptotic effect of quercetin was obtained when it was used even after the begining of ischemia, the present study could be valuable with respect to revealing the therapeutic effects of quercetin on retinal diseases, for which the pathophysiological mechanism involves I/R injury.